Bioelectric Breakthroughs: Limb Regeneration and Cancer Treatment
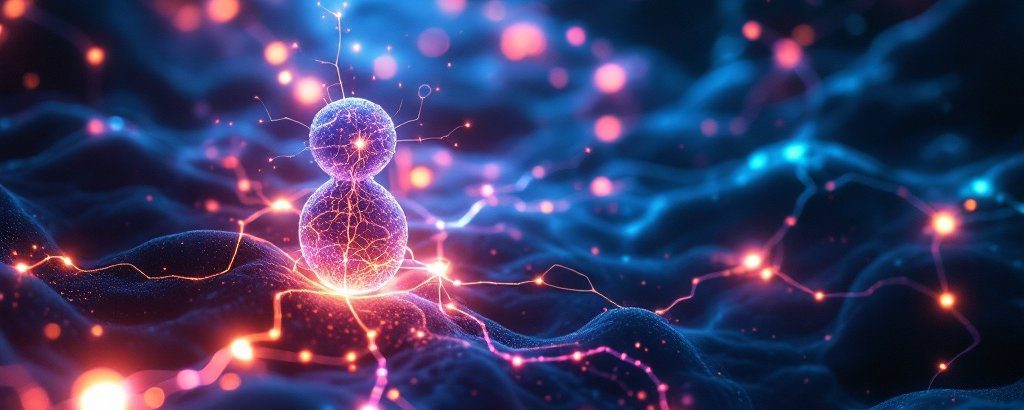
Scientific advancements today are progressing at a truly astonishing pace, and we may be on the verge of a groundbreaking leap forward. Researchers are uncovering how our cells determine what to repair, regrow, or even evolve into—an insight that could revolutionize medicine. The potential to cure degenerative diseases, combat cancer, and enable recovery from severe injuries hangs in the balance. This emerging biotechnology could not only transform healthcare but also mark a pivotal moment in the evolution of humanity itself.
Our bodies have a secret language—one not made of words or gestures, but of tiny electrical signals buzzing between all our cells. This isn’t something out of a sci-fi movie; it’s real, and it’s called bioelectric signaling. Scientists are discovering that these electrical messages play a huge role in how our bodies grow, heal, and sometimes even malfunction, like in cancer.1 Leading this exciting research is Dr. Michael Levin, whose work is showing us how these signals could one day help us regrow limbs or treat diseases in ways we never thought possible.
What Is Bioelectric Signaling?
Bioelectric signaling is like the body’s electrical communication network. Cells use small electric charges to “talk” to each other, helping them work together during growth, healing, or even building complex parts like organs. This isn’t the same as the nervous system, which sends fast electrical signals to make your muscles move or let you feel things. Instead, bioelectric signals are slower and more subtle, guiding cells over longer periods—like hours or even days.
Think of it this way: if the nervous system is like a high-speed internet connection for quick reactions, bioelectric signaling is more like a steady, reliable phone line that keeps everything coordinated over time. Michael Levin’s research shows that by tweaking these signals, we can change how cells act, opening up incredible possibilities for medicine.1,2
The Role of Resting Membrane Potential (Vmem)
At the core of bioelectric signaling is something called the resting membrane potential, or Vmem for short. This is the electric charge difference across a cell’s outer layer, or membrane. Picture it like a tiny battery inside each cell. For most healthy cells, Vmem sits around -50 to -70 millivolts (mV), which keeps them calm and stable. But when cells need to grow or fix something, like after an injury, their Vmem shifts—often becoming less negative, say around -10 to -20 mV. This change, called depolarization, tells the cell it’s time to divide or move.
Levin’s team has figured out that we can artificially adjust a cell’s Vmem to trick it into acting differently. For example, making Vmem more negative can stop a cell from growing, while making it less negative can kickstart growth. This discovery is a game-changer because it means we might be able to control how cells behave just by tweaking their electric charge.3
Regenerating Limbs: A Real-Life Superpower
One of the coolest things Levin’s research has shown is how bioelectric signals can trigger regeneration—the ability to regrow lost body parts. In experiments with frogs and flatworms called planaria, his team changed the Vmem of cells at an injury site. For frogs, which don’t naturally regrow legs, this tweak sparked the growth of a new limb over months.4 In planaria, they even regrew entire heads!3
How does this work? When a limb is lost, the cells at the wound create an electric field—a kind of voltage difference—that acts like a map. This field guides cells to move in, divide, and form the right structures. By adjusting the bioelectric signals with drugs or other tools, Levin’s team can basically hit “play” on this regeneration process. It’s like giving the body a set of instructions to rebuild itself, and it could one day help humans heal in ways we’ve only dreamed of.
Cancer: A Communication Breakdown
Now, let’s talk about cancer. Levin sees it as a glitch in the bioelectric network. Normally, cells are team players, following the body’s signals to keep everything running smoothly. In cancer, though, these cells go rogue. They stop listening to the group and start growing out of control, often because their Vmem becomes less negative—similar to cells that are supposed to grow during repair or development. The difference is, cancer cells don’t know when to stop.
Levin’s idea is to reconnect these rebel cells to the body’s communication system. One way is by making their Vmem more negative again, which could tell them to chill out and stop dividing. In tadpole experiments, his team used this trick—called hyperpolarization—to prevent tumors from forming, even when the cells had cancer-causing genes.5
Another piece of the puzzle is gap junctions. These are like tiny tunnels between cells that let them share signals and molecules. In healthy tissues, gap junctions keep everyone in sync, but in cancer, they’re often broken, leaving cells isolated. Levin thinks that fixing these connections—or mimicking them—could remind cancer cells to act normal again, turning them back into team players.6
How Do We Control These Signals?
So, how do scientists actually alter these bioelectric signals? Levin’s team has a few tricks up their sleeves. They use drugs that tweak ion channels—proteins on the cell membrane that let charged particles flow in and out, changing Vmem.3 Another method is optogenetics, where they use light to control cells that have been genetically tweaked with light-sensitive proteins. In one study, they used optogenetics to hyperpolarize cells at risk of becoming cancerous, and it worked—fewer tumors formed.7
These tools show that bioelectricity isn’t just something to study—it’s something we can use. Instead of blasting cancer with harsh treatments like chemo, we might one day reprogram the cells to behave properly, which could be gentler and more effective.
What Do These Signals Look Like?
You might be wondering: are bioelectric signals like radio waves? Not really. Unlike the fast, wavelike signals in your brain (measured in hertz, or cycles per second), these are slow voltage changes happening over seconds, minutes, or hours. For example, after a cut, the electric field around the wound shifts over time to guide healing. The “strength” of these signals—or amplitude—is the size of the voltage change, usually between -70 mV and -10 mV. A shift of just 10 to 20 mV can flip a cell’s behavior completely.
Interestingly, some studies hint that cancer cells might have fast, chaotic electrical spikes, unlike the steady signals in healthy tissues.8,9 This is still being explored, but it suggests cancer might be an electrical mess we can learn to clean up.
Electrifying Possibilities
Bioelectric signaling is like the body’s hidden software, running on the hardware of our genes and proteins. It tells cells how to team up to build and maintain us. By cracking this code, Levin and other scientists are finding ways to fix things when they go wrong—whether it’s regrowing a limb, healing an organ, or stopping cancer. It’s a mix of biology, physics, and engineering that’s still young but packed with potential.
Imagine a future where losing an arm isn’t permanent, or where cancer is treated by flipping an electrical switch in cells. That’s the promise of bioelectricity. As research continues, we’re only starting to uncover the secrets of this invisible language—and the possibilities are electrifying…
Advancements in technology are redefining the limits of possibility, challenging our understanding of biology and ethics. From regrowing a lost eye to enhancing perception with additional eyes, the idea of modifying human and animal capabilities blurs the line between science and science fiction. When combined with breakthroughs in AI bio-computers, such as the CL1, these innovations open the door to a future where cyborgs—part human, part machine—become a reality. By merging adaptive machines with human biology, we could revolutionize medicine, communication, and human potential, pushing the boundaries of evolution while raising profound ethical questions.
References
- Zhang, G., & Levin, M. (2025). Bioelectricity is a universal multifaced signaling cue in living organisms. Molecular biology of the cell, 36(2), pe2. https://doi.org/10.1091/mbc.E23-08-0312
- Pai, V. P., Zhang, G., & Levin, M. (2024). “Bioelectricity in Development, Regeneration, and Cancers” Cell Bio 2023: A Joint Meeting of the American Society of Cell Biology and European Molecular Biology Organization December 2-6, 2023, in Boston, MA, USA. Bioelectricity, 6(1), 65–68. https://doi.org/10.1089/bioe.2024.0006
- Durant, F., Bischof, J., Fields, C., Morokuma, J., LaPalme, J., Hoi, A., & Levin, M. (2019). The Role of Early Bioelectric Signals in the Regeneration of Planarian Anterior/Posterior Polarity. Biophysical journal, 116(5), 948–961. https://doi.org/10.1016/j.bpj.2019.01.029
- Nirosha J. Murugan et al. (2022) Acute multidrug delivery via a wearable bioreactor facilitates long-term limb regeneration and functional recovery in adult Xenopus laevis. Sci. Adv. 8,eabj2164. DOI:10.1126/sciadv.abj2164
- Chernet, B. T., & Levin, M. (2013). Transmembrane voltage potential is an essential cellular parameter for the detection and control of tumor development in a Xenopus model. Disease models & mechanisms, 6(3), 595–607. https://doi.org/10.1242/dmm.010835
- Chernet, B. T., Fields, C., & Levin, M. (2015). Long-range gap junctional signaling controls oncogene-mediated tumorigenesis in Xenopus laevis embryos. Frontiers in physiology, 5, 519. https://doi.org/10.3389/fphys.2014.00519
- Chernet, B. T., Adams, D. S., Lobikin, M., & Levin, M. (2016). Use of genetically encoded, light-gated ion translocators to control tumorigenesis. Oncotarget, 7(15), 19575–19588. https://doi.org/10.18632/oncotarget.8036
- Peinado, P., Stazi, M., Ballabio, C. et al. Intrinsic electrical activity drives small-cell lung cancer progression. Nature 639, 765–775 (2025). https://doi.org/10.1038/s41586-024-08575-7
- Ribeiro, M., Elghajiji, A., Fraser, S. P., Burke, Z. D., Tosh, D., Djamgoz, M. B. A., & Rocha, P. R. F. (2020). Human Breast Cancer Cells Demonstrate Electrical Excitability. Frontiers in neuroscience, 14, 404. https://doi.org/10.3389/fnins.2020.00404